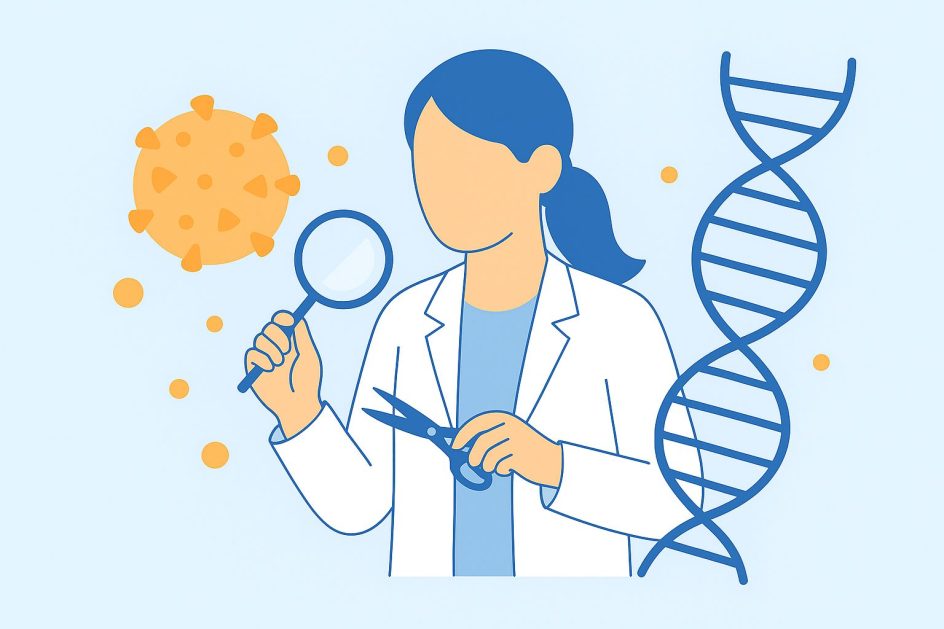
Uncovering the Genetic Secrets Behind Cholesterol with CRISPR Technology
Cholesterol plays an essential role in our bodies, but too much of it can lead to serious health issues. This project explores how genetic changes contribute to cholesterol uptake by our cells.
Cardiovascular diseases, such as coronary artery disease, heart failure, high blood pressure, and stroke, are the leading cause of death worldwide (Di Cesare et al., 2024). The key driver is atherosclerosis, the process of narrowing of arteries due to buildup of substances on the arterial walls (Libby et al., 2019). High cholesterol levels in the bloodstream have been associated with a higher risk of cardiovascular diseases, since this molecule is a major contributor to atherosclerosis (Ryu et al., 2024).
The risk of heart conditions is mostly influenced by environmental factors, including smoking and diet. However, genetic variations in cholesterol metabolism also play a role (Hamilton et al., 2023). For instance, familiar hypercholesterolemia is a genetic disorder caused by inherited mutations in cholesterol-related genes, leading to elevated levels of circulating LDL cholesterol and an increased risk of cardiovascular diseases (Duan et al., 2022). Consequently, scientific studies, including this project, focus on identification of strategies to effectively lower LDL cholesterol. But why does this small molecule get some much attention in the first place?
Cholesterol is a fat-like substance present in our blood and cells that is crucial for building cells and making hormones. It can either be synthesized in the body, which happens mostly in the liver, or it is obtained from the diet (Duan et al., 2022). There are two main types – the bad cholesterol, LDL, which delivers cholesterol from the liver to other tissues, and the good cholesterol, HDL, which collects excess cholesterol from the bloodstream and brings it back to the liver for excretion (Duan et al., 2022). These two are the major players in cholesterol transport – but how do we uncover the genes that control them? Thanks to CRISPR, scientists can alter these genes and discover new solutions for heart health.
CRISPR and base editing
All the genetic information in our body is stored in DNA, and gene editing tools provide an efficient way to alter this information (Anzalone et al., 2020). For this purpose, CRISPR-based technologies are widely used, since they allow for precise modification, removal, or replacement of genetic sequences (Hess et al., 2017). CRISPR provides various tools to edit the genome, including base editing (Banerjee & Sherwood, 2017), which was applied in this project.
Instead of cleaving the DNA like more traditional CRISPR-based technologies, base editors enable the replacement of a single DNA base with another at a specific location in the genome (Anzalone et al., 2020). Therefore, base editors are characterized by high precision and minimal mutation. In this experiment, we used a base editor Sdd7, which has been recently discovered through an AI-based approach (Huang et al., 2023). Thanks to CRISPR and AI, modern science and medicine have entered a whole new era of possibilities.
Results
The aim of the project was to investigate which edits create the most outstanding impact on cholesterol uptake. Firstly, around 2500 variants within 18 genomic regions were chosen based on previously generated data. Next, using base editing, these variants were introduced into liver cells, followed by the addition of fluorescent LDL. Cholesterol uptake was evaluated based on the fluorescence response of each variant – this showed which edits increase the uptake and could be potential therapeutic targets, and which decrease it and could possibly elevate the risk of cardiovascular diseases.
Out of the 2493 variants tested, 657 were identified as significant. These will be further investigated in relation to cholesterol through different sets of bioinformatics pipelines and laboratory experiments, with the aim of pinpointing the most significant ones. As far as the current project goes, top 20 responses were examined. All of these variants were in proximity to cholesterol-related genes, which makes perfect sense since they showed an effect on LDL uptake. But before any major conclusions can be drawn, there is still more work to do – follow-up experiments are a must!
Key takeaways
am very grateful to have had the chance to work on this project at a lab at Brigham and Women’s Hospital and Harvard Medical School. Boston is the biotech hub of the world, filled with top-tier academics and endless opportunities. Through daily lab work, I have grown as a scientist – I have not only learned various laboratory techniques, data analysis methods, and scientific skills, but also had the opportunity to manage a project independently, which has been a wonderful learning experience. Additionally, during this time, I expanded my knowledge of CRISPR, gene modification, and gene regulation. Most importantly, through my project, I have contributed to advancements in science, and I want to sincerely thank everyone who made this possible.
References
Anzalone, A. V., Koblan, L. W., & Liu, D. R. (2020). Genome editing with CRISPR–Cas nucleases, base editors, transposases and prime editors. Nature Biotechnology 2020 38:7, 38(7), 824–844. https://doi.org/10.1038/s41587-020-0561-9
Banerjee, B., & Sherwood, R. I. (2017). A CRISPR view of gene regulation. Current Opinion in Systems Biology, 1, 1–8. https://doi.org/10.1016/J.COISB.2016.12.016
Di Cesare, M., McGhie, D. V., Perel, P., Mwangi, J., Taylor, S., Pervan, B., Kabudula, C., Narula, J., Bixby, H., Pineiro, D., Gaziano, T. A., & Pinto, F. J. (2024). The Heart of the World. Global Heart, 19(1), 11. https://doi.org/10.5334/GH.1288
Duan, Y., Gong, K., Xu, S., Zhang, F., Meng, X., & Han, J. (2022). Regulation of cholesterol homeostasis in health and diseases: from mechanisms to targeted therapeutics. Signal Transduction and Targeted Therapy 2022 7:1, 7(1), 1–29. https://doi.org/10.1038/s41392-022-01125-5
Hamilton, M. C., Fife, J. D., Akinci, E., Yu, T., Khowpinitchai, B., Cha, M., Barkal, S., Thi, T. T., Yeo, G. H. T., Ramos Barroso, J. P., Francoeur, M. J., Velimirovic, M., Gifford, D. K., Lettre, G., Yu, H., Cassa, C. A., & Sherwood, R. I. (2023). Systematic elucidation of genetic mechanisms underlying cholesterol uptake. Cell Genomics, 3(5), 100304. https://doi.org/10.1016/J.XGEN.2023.100304
Hess, G. T., Tycko, J., Yao, D., & Bassik, M. C. (2017). Methods and Applications of CRISPR-Mediated Base Editing in Eukaryotic Genomes. Molecular Cell, 68(1), 26–43. https://doi.org/10.1016/J.MOLCEL.2017.09.029
Huang, J., Lin, Q., Fei, H., Qiu, J.-L., Zhao, K. T., & Gao Correspondence, C. (2023). Discovery of deaminase functions by structure-based protein clustering. Cell, 186, 3182-3195.e14. https://doi.org/10.1016/j.cell.2023.05.041
Libby, P., Buring, J. E., Badimon, L., Hansson, G. K., Deanfield, J., Bittencourt, M. S., Tokgözoğlu, L., & Lewis, E. F. (2019). Atherosclerosis. Nature Reviews Disease Primers 2019 5:1, 5(1), 1–18. https://doi.org/10.1038/s41572-019-0106-z
Ryu, J., Barkal, S., Yu, T., Jankowiak, M., Zhou, Y., Francoeur, M., Phan, Q. V., Li, Z., Tognon, M., Brown, L., Love, M. I., Bhat, V., Lettre, G., Ascher, D. B., Cassa, C. A., Sherwood, R. I., & Pinello, L. (2024). Joint genotypic and phenotypic outcome modeling improves base editing variant effect quantification. Nature Genetics, 56(5), 925–937. https://doi.org/10.1038/s41588-024-01726-6